Abstract
Severe pollution environment over the Israel Electric (IECo) Power Grid is created by synergy between Saharan dust and sea-salt aerosols (SSA) originated from the Mediterranean Sea. In the presence of high air humidity, the resultant pollution stress remains one of the major factors affecting operational reliability of ceramic insulators in the IECo Network. In respect with the severe polluted conditions, the outdoor insulation coordination requires detailed statistical studies and refined geographic mapping of the natural pollution deposit. This paper presents numeric maps of Sea-Salt Sedimentation Rate (SSSR) that was developed for the Eastern Mediterranean region including Israel. The paper demonstrates an adopted meteorological model, calculating algorithm and spatial–temporal characteristics of sea-salt aerosols within the considered domain. The acquired SSSR-Maps have been verified against the Equivalent Salt Deposit Density (ESDD) field-data and available ground-level measurements of SSA-concentrations. Good agreement was obtained between SSA model predictions and ground-based measurements on a daily and monthly basis. A case-study of a joint analysis of desertdust and sea-salt deposition along the selected overhead line was presented. The effect of the sea-salt deposit on the pollution flashover risk of ceramic insulators has been demonstrated. It is showed that the predicted ESDD-values might quite commensurate those ESDD-portions absorbed by desert dust. Hence, the contribution of sea-salt deposit cannot be neglected when evaluating pollution flashover risk for local outdoor insulators, especially in coastal areas. The study outcomes have demonstrated that the proposed SSA-modeling approach has sufficient meaningfulness to be implemented in practical tasks of outdoor insulation coordination and insulators dimensioning.












Similar content being viewed by others
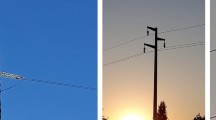
References
Volpov E, Kishcha P (2017) An advanced technique for outdoor insulation pollution mapping in the Israel electric company power grid. IEEE Trans Dielectr Electr Insul 24(6):3539–3548
Volpov E, Linder R, Kishcha P (2018) Development of outdoor insulation pollution maps for IECo Power Grid. CIGRE Session 2018, Paris, paper C4-201
Kishcha P, Volpov E, Starobinets B, Alpert P, Nickovic S (2020) Dust dry deposition over Israel. Atmosphere 11(2):1–17
Rizk F, El-Arbaty A, El-Sarky A (1975) Laboratory and field experiences with the EHV transmission line insulators in the desert. IEEE Trans Power App Syst 94(5):1770–1776
(2000) Polluted insulators: a review of current knowledge. CIGRE Technical Brochure 158, Task Force 33.04.01, Paris
(2008) Outdoor insulation in polluted conditions: guidelines for selection and dimensioning. CIGRE TB-361, WG C4.303
(2008) IEC TC 60815-1, selection and dimensioning of high-voltage insulators intended for use in polluted conditions, part-I: definitions, information and general principles, 1st edn
Rizk F (1981) Mathematical Models for pollution flashover. Electra 78:71–103
Randles CA, da Silva AM, Buchard V, Colarco PR, Darmenov A, Govindaraju R, Smirnov A, Holben B, Ferrare R, Hair J, Shinozuka Y, Flynn CJ (2017) The MERRA-2 aerosol reanalysis, 1980 onward. Part I: system description and data assimilation evaluation. J Clim 30:6823–6850
Blanchard DC (1983) The production, distribution, and bacterial enrichment of the sea-salt aerosol. In: Liss P, Slinn W (eds) Air-sea exchange of gases and particle. Springer, Berlin, pp 407–454
Lewis ER, Schwartz SE (2004) Sea salt aerosol production: mechanisms, methods, measurements, and models—a critical review. AGU, Washington, DC
De Leeuw G, Andreas EL, Anguelova MD, Fairall CW, Lewis ER, C ODowd, M Schulz, SE Schwartz, (2001) Production flux of sea spray aerosol. Rev Geophys. https://doi.org/10.1029/2010RG000349
Clarke AD, Owens SR, Zhou J (2006) An ultrafine sea-salt flux from breaking waves: Implications for cloud condensation nuclei in the remote marine atmosphere. J Geophys Res 111:D06202. https://doi.org/10.1029/2005JD006565
(1999) Natural and artificial ageing and pollution testing of polymeric insulators. CIGRE Technical Brochure 142, Task Force 33.04.07. Paris
(2005) IEEE Std. C62.11, Metal Oxide Surge Arresters for AC Power Circuits (>1 kV)
Tyler RH, Boyer TP, Minami T et al (2017) Electrical conductivity of the global ocean. Earth Planets Space 69:156. https://doi.org/10.1186/s40623-017-0739-7
Kishcha P, Nickovic S, Starobinets B, di Sarra A, Udisti R, Becagli S, Sferlazzo D, Bommarito C, Alpert P (2011) Sea-salt aerosol forecasts compared with daily measurements at the island of Lampedusa (Central Mediterranean). Atmos Res 100(1):28–35
Nickovic S, Janjic ZI, Kishsha P, Alpert P (2007) Model for simulation of the sea salt aerosol atmospheric cycle. Res Act Atmos Oceanic Model 20:19–20
Nickovic S, Kallos G, Papadopoulos A, Kakaliagou O (2001) A model for prediction of desert dust cycle in the atmosphere. J Geophys Res 106:18113–18130
Mesinger F, Janjik Z, Nickovic S, Gavrilov D, Deaven D (1988) The step-mountain coordinate: model description and performance for cases of Alpine lee cyclogenesis and for a case of an Appalachian redevelopment. Mon Weather Rev 116:1493–1505
Janjic ZI (1994) The step-mountain eta coordinate model: further developments of the convection, viscous sublayer and turbulence closure schemes. Mon Weather Rev 122:927–945
Tecora Skypost PM FilterGuard, available at: www.tcr-tecora.com
Nessim R, Tadros H, Taleb A, Moawad M (2015) Chemistry of the Egyptian Mediterranean coastal waters. Egyp J Aquatic Res 41:1–10. https://doi.org/10.1016/j.ejar.2015.01.004
Water salinity in the Mediterranean Se ais 40 ‰ (g/kg). https://en.wikipedia.org/wiki/List_of_bodies_of_water_by_salinity
NCEP Global Forecast System. www.emc.ncep.noaa.gov/index.php?branch=GFS
Georgi F (1986) A particle dry-deposition parameterization scheme for use in tracer transport models. J Geophys Res 91:9794–9806
GRADS: The Grid Analysis and Display System. https://www.cpc.ncep.noaa.gov/products/wesley/links_grads.html
Acknowledgements
The authors gratefully acknowledge the contribution of their colleagues Dr. R. Linder and Dr. D. Zinemanas (IECo) for their valuable work on GIS application and chemical tests.
Author information
Authors and Affiliations
Corresponding author
Additional information
Publisher's Note
Springer Nature remains neutral with regard to jurisdictional claims in published maps and institutional affiliations.
Appendices
Appendix A
1.1 Chemical structure of the local SSA and sea-water
The chemical structure of sea-salt particles is similar to that of sea-water. According to Nessim et al. [23], the following six major ions: Na + , Cl-, SO4-2, Mg + 2, Ca + 2 and K + , make up > 99% of the total dissolved salts in the Eastern Mediterranean Sea-water. Based on our long-term measurements, Table 2 presents typical chemical components of the coastal sea-water.
The representative mass-relations, as per Table 2 can be characterized as very stable, which have fairly low monthly and directional variations along the local 230-km-shoreline. The data from Table 2 enable direct evaluation of the key-index γ that represents specific weight of NaCl in the total mass of sea-salts (see Eq. (4)). As per our estimates, the γ-index can be reliably assigned to be 0.85 for local conditions. The latter ratio causes a respective very high salinity (~ 40-g/kg) of the Eastern Mediterranean Sea-water which is one of the most saline sea areas in the world [24].
Appendix B
2.1 The ‘Dream-Salt’ model
Below we will briefly explain a principal Flowchart, as per Fig. 13, which depicts the involved modules, data-exchange and calculating algorithm.
At the 1-st step, the NCEP/Eta meteorological model is initialized with the NCEP analysis [20, 25]. Boundary conditions are updated every 6 h by the NCEP Global Forecast System [25] meteorological model (Fig. 13, PREPROCESSING).
At the 2-nd step, the Eta (η) meteorological model [20, 21] solves the system of hydrodynamic equations as per (B1)–(B9), which describe basic meteorological parameters, such as winds, temperature, pressure, etc. (Fig. 13, ATMOSPHERIC MODELING).
Here v is the horizontal velocity vector, f is the Coriolis parameter, k is the vertical unit vector, Φ is geopotential, R is the gas constant, and k is R/CP, where CP is the specific heat at constant pressure. Eta uses the η-vertical coordinate written as [20, 21]:
where p is pressure; the subscripts T and S stand for the top and the ground surface values of the model atmosphere, respectively; Z is geometric height, and prf (Z) is a reference pressure as a function of Z. The η-coordinate improves the calculation of horizontal derivatives near steep topographic areas.
At the 3-rd step, the DREAM Sea-salt module is initialized with 3-D sea-salt distributions obtained from previous sea-salt forecasts (i.e., previous model runs) (Fig. 13, SEA-SALT MODELING). Each run-session starts at 12:00 UTC and sea-salt predictions were performed for 6-h periods up to 72 h ahead. The sea-salt module of DREAM-Salt solves the Euler-type partial differential nonlinear equations for sea-salt mass continuity. The Eulerian concentration equation is given by the following expression:
where k indicates the number of particle size classes; Ck is the sea-salt concentration of a k-th particle size class; u and ν are components of horizontal wind velocity; w is the vertical velocity; KH is the lateral diffusion coefficient, KZ is the turbulent exchange coefficient. νgk is the gravitational settling velocity, SINK is the sink term which includes both wet and dry deposition [26]. SOURCE is meaning sea-salt emissions. Eight size bins covering the particle effective sizes from 0.1 μm to 7 μm are used in the model.
At the 4-th step, the DREAM-Salt output files are created (Fig. 13, POSTPROCESSING). The Grid Analysis and Display System Software (GRADS) has been used for creating DREAM-Salt output files [27]. The output files contain space–time distribution of sea-salt concentration/deposition over the modeled domain for every six hours, starting from 12:00 UTC and up to 72 h ahead.
At the 5-th step, the DREAM-Salt output files that were generated in the GRADS format are now compressed and archived in the Multi-Year Archive of SSA-deposition over the modeled domain (Fig. 13, Multi-Year ARCHIVE).
The last 6-th step of the modeling procedure (Fig. 13, MAPPING) is described in Chapter 4.
Rights and permissions
About this article
Cite this article
Volpov, E., Kishcha, P. Advanced modeling and mapping of severe pollution stress required for outdoor insulation coordination. Electr Eng 104, 741–752 (2022). https://doi.org/10.1007/s00202-021-01333-2
Received:
Accepted:
Published:
Issue Date:
DOI: https://doi.org/10.1007/s00202-021-01333-2