Abstract
Since the advent of the commercial da Vinci surgical system, microsurgical robotic systems have been increasingly investigated. These systems improve the accuracy and dexterity of surgeries using a computerized motion scaling function between a surgeon’s hand and surgical instrument. However, the manufacturing process of these systems is expensive owing to the use of multiple sensors, actuators, and controllers. Additionally, certain limitations exist, such as an increase in the size of the operating room to accommodate the robotic system and the absence of tactile force feedback. To address these problems, we propose a passive motion scaling mechanism for microsurgery. Based on the pantograph mechanism, we downscale the linear displacement of the surgeon’s hand and transfer it to the surgical instrument. Furthermore, the rotational displacement of the surgeon’s hand in an actual surgery is transmitted to the surgical instrument on a 1:1 scale by applying a parallelogram mechanism. We designed and fabricated a prototype capable of transmitting linear and rotational displacements (pitch and yaw) of the surgeon’s hand with three degrees of freedom (DOFs) and two DOFs, respectively, by combining the two mechanisms. The obtained experimental results verify the motion scaling function of the developed prototype.














Similar content being viewed by others
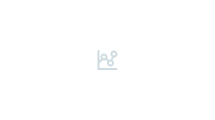
References
Kazmitcheff, G., Miroir, M., Nguyen, Y., Célérier, C., Mazalaigue, S., Ferraty, E., Sterkers, O., & Grayeli, A. B. (2011). Evaluation of command modes of an assistance robot for middle ear surgery. In Proc. of IEEE/RSJ international conference on intelligent robots and systems (pp. 850–856).
Simaan, N., Bajo, A., Reiter, A., Wang, L., Kazanzides, P., Allen, P., & Fowler, D. (2013). Lessons learned using the insertable robotic effector platform (IREP) for single port access surgery. Journal of Robotics Surgery, 7, 235–240.
Boyd, W. D., Rayman, R., Desai, N. D., et al. (2000). Closed-chest coronary artery bypass grafting on the beating heart with the use of a computer-enhanced surgical robotic system. The Journal of Thoracic and Cardiovasc Surgery, 120, 807–809.
Simaan, N., Taylor, R., Flint, P., Chirikjian, G., & Stein, D. (2013). Devices, systems and methods for minimally invasive surgery of the throat and other portions of mammalian body. US Patent, US8365633B2.
Choi, D. E., Kim, S. H., Lee, W. S., Kang, S. C., & Kim, K. R. (2021). Development and preclinical trials of a surgical robot system for endoscopic endonasal transsphenoidal surgery. International Journal of Control, Automation and Systems, 19, 1–11.
Huynh, L. M., & Kim, Y. H. (2013). A computer-aided and robot-assisted surgical system for reconstruction of anterior cruciate ligament. International Journal of Precision Engineering and Manufacturing, 14(1), 49–54.
Lanfranco, A. R., Castellanos, A. E., Desai, J. P., & Meyers, W. C. (2018). Robotic surgery: a current perspective. Annals of Surgery, 239(1), 14.
Tan, Y., Liverneaux, P., & Wong, J. K. (2018). Current limitations of surgical robotics in reconstructive plastic microsurgery. Frontiers in Surgery, 5(22), 1–9.
Roizenblatt, M., Edwards, T. L., & Gehlbach, P. L. (2018). Robot-assisted vitreoretinal surgery: Current perspectives. Robotic Surgery: Research and Reviews, 5, 1.
Magdy, M., Fanni, M., Mohamed, A. M., & Miyashita, T. (2017). Kinematic design and novel mobility analysis of a new 3d pantograph decoupled manipulator. Mechanism and Machine Theory, 117, 253–275.
Briot, S., & Bonev, I. A. (2019). Pantopteron: A new fully decoupled 3DOF translational parallel robot for pick-and-place applications. Journal of Mechanisms and Robotics, 1(2), 021001.
Briot, S., & Bonev, I. A. (2010). Pantopteron-4: A new 3T1R decoupled parallel manipulator for pick-and-place applications. Mechanism and Machine Theory, 45(5), 707–721.
Elgammal, A. T., Fanni, M., & Mohamed, A. M. (2017). Design and analysis of a novel 3d decoupled manipulator based on compliant pantograph for micromanipulation. Journal of Intelligent & Robotic Systems, 87(1), 43–57.
Lee, T.-H., Choi, D., & Lee, W. (2021). Computational design and workspace analysis of a passive motion-scaling mechanism based on pantograph for microsurgery. Journal of Computational Design and Engineering, 8(6), 1446–1467.
Das, H., Zak, H., Johnson, J., Crouch, J., & Frambach, D. (1999). Evaluation of a telerobotic system to assist surgeons in microsurgery. Computer Aided Surgery, 4(1), 15–25.
Maddahi, Y., Gan, L. S., Zareinia, K., Lama, S., Sepehri, N., & Sutherland, G. R. (2016). Quantifying workspace and forces of surgical dissection during robot-assisted neurosurgery. The International Journal of Medical Robotics and Computer Assisted Surgery, 12(3), 528–537.
Zhang, D., Chen, J., Li, W., Salinas, D. B., & Yang, G. Z. (2020). A microsurgical robot research platform for robot-assisted microsurgery research and training. International Journal of Computer Assisted Radiology and Surgery, 15(1), 15–25.
Prasad, S. M., Prasad, S. M., Maniar, H. S., Chu, C., Schuessler, R. B., & Damiano, R. J., Jr. (2004). Surgical robotics: Impact of motion scaling on task performance. Journal of the American College of Surgeons, 199(6), 863–868.
Salcudean, S. E., Ku, S., & Bell, G. (1997). Performance measurement in scaled teleoperation for microsurgery. In First joint conference computer vision, virtual reality and robotics in medicine and medical robotics and computer-assisted surgery (CVRMed-MRCAS'97) (pp. 789–798).
Cassilly, R., Diodato, M. D., Bottros, M., & Damiano, R. J., Jr. (2004). Optimizing motion scaling and magnification in robotic surgery. Surgery, 136(2), 291–294.
Acknowledgements
This work was supported by the Korea Institute of Science and Technology (KIST) Institutional Program under Grant No. 2E31072.
Author information
Authors and Affiliations
Corresponding authors
Additional information
Publisher's Note
Springer Nature remains neutral with regard to jurisdictional claims in published maps and institutional affiliations.
Jae-Bok Song and Woosub Lee have contributed equally to this work.
Rights and permissions
About this article
Cite this article
Choi, D., Lee, TH., Song, JB. et al. Design and Validation of a Passive Motion Scaling Mechanism Prototype for Microsurgery. Int. J. Precis. Eng. Manuf. 23, 1065–1075 (2022). https://doi.org/10.1007/s12541-022-00624-3
Received:
Revised:
Accepted:
Published:
Issue Date:
DOI: https://doi.org/10.1007/s12541-022-00624-3