Abstract
Background:
Three-dimensional (3D) in vitro cultures recapitulate the physiological microenvironment and exhibit high concordance with in vivo conditions. Improving co-culture models with different kind of cell types cultured on a 3D scaffold can closely mimic the in vivo environment. In this study, we examined the osteogenic response of pre-osteoblast MC3T3-E1 cells and Raw264.7 mouse monocytes in a 3D-encapsulated co-culture environment composed of the Cellrix® 3D culture system, which provides a physiologically relevant environment.
Methods:
The Cellrix® 3D Bio-Gel scaffolds were used to individually culture or co-culture two type cells in 3D microenvironment. Under 3D culture conditions, osteoblastic behavior was evaluated with an ALP assay and staining. ACP assay and TRAP staining were used as osteoclastic behavior indicator.
Results:
Treatment with osteoblastic induction factors (+3F) and RANKL had on positively effect on alkaline phosphatase activity but significantly inhibited to acid phosphatase activity during osteoclastic differentiation in 3D co-culture. Interestingly, alkaline phosphatase activity or acid phosphatase activity in 3D co-culture was stimulated with opposite differentiation factors at an early stage of differentiation. We guess that these effects may be related to RANK–RANKL signaling, which is important in osteoblast regulation of osteoclasts.
Conclusion:
In this study, the osteogenic response of 3D encapsulated pre-osteoblast MC3T3-E1 cells and mouse monocyte Raw264.7 cells was successfully demonstrated. Our 3D culture conditions will be able to provide a foundation for developing a high-throughput in vitro bone model to study the effects of various drugs and other agents on molecular pathways.









Similar content being viewed by others
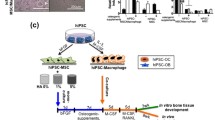
References
Rijal G, Bathula C, Li W. Application of synthetic polymeric scaffolds in breast cancer 3D tissue cultures and animal tumor models. Int J Biomater. 2017;2017:8074890.
Torricelli P, Fini M, Giavaresi G, Giardino R. In vitro models to test orthopedic biomaterials in view of their clinical application in osteoporotic bone. Int J Artif Organs. 2004;27:658–63.
Chinta MS, des Jardins-Park HE, Wan DC, Longaker MT. “Tissues in a dish”: a review of organoids in plastic surgery. Plast Reconstr Surg Glob Open. 2020;8:e2787.
Elliott NT, Yuan F. A review of three-dimensional in vitro tissue models for drug discovery and transport studies. J Pharm Sci. 2011;100:59–74.
Huang G, Li F, Zhao X, Ma Y, Li Y, Lin M, et al. Functional and biomimetic materials for engineering of the three-dimensional cell microenvironment. Chem Rev. 2017;117:12764–850.
Gevaert M. Engineering 3D tissue systems to better mimic human biology. Bridge. 2012;42:48–55.
Weigelt B, Lo AT, Park C, Gray JW, Bissell MJ. HER2 signaling pathway activation and response of breast cancer cells to HER2-targeting agents is dependent strongly on the 3D microenvironment. Breast Cancer Res Treat. 2010;122:35–43.
Sieni E, Dettin M, De Robertis M, Bazzolo B, Conconi MT, Zamuner A, et al. The efficiency of gene electrotransfer in breast-cancer cell lines cultured on a novel collagen-free 3D scaffold. Cancers (Basel). 2020;12:1043.
Prestwich GD. Simplifying the extracellular matrix for 3-D cell culture and tissue engineering: a pragmatic approach. J Cell Biochem. 2007;101:1370–83.
Sharma A, Lee MG, Won M, Koo S, Arambula JF, Sessler JL, et al. Targeting heterogeneous tumors using a multifunctional molecular prodrug. J Am Chem Soc. 2019;141:15611–8.
Koo S, Bobba KN, Cho MY, Park HS, Won M, Velusamy N, et al. Molecular theranostic agent with programmed activation for hypoxic tumors. ACS Appl Bio Mater. 2019;2:4648–55.
Song HK, Noh EM, Kim JM, You YO, Kwon KB, Lee YR. Reversine inhibits MMP-3, IL-6 and IL-8 expression through suppression of ROS and JNK/AP-1 activation in interleukin-1β-stimulated human gingival fibroblasts. Arch Oral Biol. 2019;108:104530.
Cukierman E, Pankov R, Stevens DR, Yamada KM. Taking cell-matrix adhesions to the third dimension. Science. 2001;294:1708–12.
Bokhari M, Carnachan RJ, Cameron NR, Przyborski SA. Culture of HepG2 liver cells on three dimensional polystyrene scaffolds enhances cell structure and function during toxicological challenge. J Anat. 2007;211:567–76.
Sethi T, Rintoul RC, Moore SM, MacKinnon AC, Salter D, Choo C, et al. Extracellular matrix proteins protect small cell lung cancer cells against apoptosis: a mechanism for small cell lung cancer growth and drug resistance in vivo. Nat Med. 1999;5:662–8.
Prestwich GD. Evaluating drug efficacy and toxicology in three dimensions: using synthetic extracellular matrices in drug discovery. Acc Chem Res. 2008;41:139–48.
Labarbera DV, Reid BG, Yoo BH. The multicellular tumor spheroid model for high-throughput cancer drug discovery. Expert Opin Drug Discov. 2012;7:819–30.
Davidenko N, Schuster CF, Bax DV, Farndale RW, Hamaia S, Best SM, et al. Evaluation of cell binding to collagen and gelatin: a study of the effect of 2D and 3D architecture and surface chemistry. J Mater Sci Mater Med. 2016;27:148.
Han SH, Cha M, Jin YZ, Lee KM, Lee JH. BMP-2 and hMSC dual delivery onto 3D printed PLA-Biogel scaffold for critical-size bone defect regeneration in rabbit tibia. Biomed Mater. 2020. https://doi.org/10.1088/1748-605X/aba879.
Czekanska EM, Stoddart MJ, Richards RG, Hayes JS. In search of an osteoblast cell model for in vitro research. Eur Cell Mater. 2012;24:1–17.
Quarles LD, Yohay DA, Lever LW, Caton R, Wenstrup RJ. Distinct proliferative and differentiated stages of murine MC3T3-E1 cells in culture: an in vitro model of osteoblast development. J Bone Miner Res. 1992;7:683–92.
Tevlek A, Hosseinian P, Ogutcu C, Turk M, Aydin HM. Bi-layered constructs of poly(glycerol-sebacate)-β-tricalcium phosphate for bone-soft tissue interface applications. Mater Sci Eng C Mater Biol Appl. 2017;72:316–24.
Hotokezaka H, Sakai E, Kanaoka K, Saito K, Matsuo K, Kitaura H, et al. U0126 and PD98059, specific inhibitors of MEK, accelerate differentiation of RAW264.7 cells into osteoclast-like cells. J Biol Chem. 2002;277:47366–72.
Roeder I, Loeffler M, Glauche I. Towards a quantitative understanding of stem cell-niche interaction: experiments, models, and technologies. Blood Cells Mol Dis. 2011;46:308–17.
Mercatali L, Spadazzi C, Miserocchi G, Liverani C, De Vita A, Bongiovanni A, et al. Development of a human preclinical model of osteoclastogenesis from peripheral blood monocytes co-cultured with breast cancer cell lines. J Vis Exp. 2017;127:56311.
Penolazzi L, Lolli A, Sardelli L, Angelozzi M, Lambertini E, Trombelli L, et al. Establishment of a 3D-dynamic osteoblasts–osteoclasts co-culture model to simulate the jawbone microenvironment in vitro. Life Sci. 2016;152:82–93.
Iwamoto Y, Nishikawa K, Imai R, Furuya M, Uenaka M, Ohta Y, et al. Intercellular communication between keratinocytes and fibroblasts induces local osteoclast differentiation: a mechanism underlying cholesteatoma-induced bone destruction. Mol Cell Biol. 2016;36:1610–20.
Kang YK, Zhang MC. IL-23 promotes osteoclastogenesis in osteoblast–osteoclast co-culture system. Genet Mol Res. 2014;13:4673–9.
Mukherjee A, Rotwein P. Selective signaling by Akt1 controls osteoblast differentiation and osteoblast-mediated osteoclast development. Mol Cell Biol. 2012;32:490–500.
Yao Z, Xing L, Qin C, Schwarz EM, Boyce BF. Osteoclast precursor interaction with bone matrix induces osteoclast formation directly by an interleukin-1-mediated autocrine mechanism. J Biol Chem. 2008;283:9917–24.
Jones GL, Motta A, Marshall MJ, El Haj AJ, Cartmell SH. Osteoblast: osteoclast co-cultures on silk fibroin, chitosan and PLLA films. Biomaterials. 2009;30:5376–84.
Orlandini SZ, Formigli L, Benvenuti S, Lasagni L, Franchi A, Masi L, et al. Functional and structural interactions between osteoblastic and preosteoclastic cells in vitro. Cell Tissue Res. 1995;281:33–42.
Acknowledgements
This research was supported by the Basic Science Research Program through the National Research Foundation of Korea (NRF), funded by the Ministry of Education, Science and Technology (NRF-2018R1D1A1B07040482) and by Grant No. 03-2016-0190 from the SNUH Research Fund.
Author information
Authors and Affiliations
Corresponding authors
Ethics declarations
Conflict of interest
The authors declare that they have no conflict of interest.
Ethical statement
There are no animal experiments carried out for this article.
Additional information
Publisher's Note
Springer Nature remains neutral with regard to jurisdictional claims in published maps and institutional affiliations.
Electronic supplementary material
Below is the link to the electronic supplementary material.
Rights and permissions
About this article
Cite this article
Kim, J., Lyu, HZ., Jung, C. et al. Osteogenic Response of MC3T3-E1 and Raw264.7 in the 3D-Encapsulated Co-Culture Environment. Tissue Eng Regen Med 18, 387–397 (2021). https://doi.org/10.1007/s13770-020-00321-0
Received:
Revised:
Accepted:
Published:
Issue Date:
DOI: https://doi.org/10.1007/s13770-020-00321-0