Abstract
The mechanisms by which thermocapillary convection arises during boiling of nominally pure fluids in low-g environments are currently not known. It has recently been suggested that small amounts of dissolved gas within the bulk liquid can accumulate within the vapor bubble, forming localized concentration gradients that results in a temperature gradient to form along the liquid–vapor interface that drives thermocapillary convection. This hypothesis was tested by boiling > 99.3% pure n-perfluorohexane with and without noncondensible gas in a low-g environment using a 7.0 × 7.0 mm2 microheater array to measure time and space resolved heat transfer at various wall superheats. The thermocapillary convection around the primary bubble that formed in the gassy fluid was found to be much weaker than in the degassed fluid, and the primary bubble diameter was much larger in the gassy fluid due to the accumulation of noncondensible gas within the bubble. The results suggest that the accumulation of noncondensible gas in the bubble can result in temperature variations along the interface but due to the increased vapor/gas bubble size, the driving thermocapillary temperature gradient along the interface is significantly reduced and result in much weaker thermocapillary flow. The highest CHF values in a reduced gravity environment (19 W/cm2) occurred when the fluid was highly subcooled and degassed.






Similar content being viewed by others
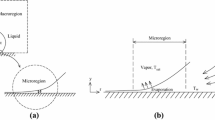
References
Abe Y, Iwasaki A (1999) Single and dual vapor bubble experiments in microgravity. In: Proceeding of the microgravity fluid physics and heat transfer conference, September 19–24, Kahuku, Hawaii
Abe Y, Oka T, Mori MH, Nagashima A (1994) Pool boiling of non-azeotropic binary mixture under microgravity. Int J Heat Mass Transf 37:2405–2414
Ahmed S, Carey VP (1998) Effects of gravity on the boiling of binary fluid mixtures. Int J Heat Mass Transf 41(16):2469–2483
Bae S, Kim MH, Kim J (1999) Improved technique to measure time and space resolved heat transfer under single bubbles during saturated pool boiling of FC-72. Exp Heat Transf 12(3):265–278
Di Marco P (2003) Review of reduced gravity boiling heat transfer: European research. Jpn Soc Microgravity Appl J 20(4):252–263
Henry CD, Kim J (2005) Thermocapillary effects on low-g pool boiling from microheater arrays of various aspect ratio. Microgravity Sci Technol XVI:170–175
Henry CD, Kim J, Chamberlain B, Hartmann TG (2005) Heater aspect ratio effects on pool boiling heat transfer under varying gravity conditions. Exp Therm Fluid Sci 29(7):773–782
Ivey H, Morris DJ (1962) On the relevance of the vapor–liquid exchange mechanism for subcooled boiling heat transfer at high pressure, British Rep. AEEW-R-137, Atomic Energy Establishment, Winfrith
Kao YS, Kenning DBR (1972) Thermocapillary flow near a hemispherical bubble on a heater wall. J Fluid Mech 53:715–735
Kim J (2003) Review of reduced gravity boiling heat transfer: US research. Jpn Soc Microgravity Appl J 20(4):264–271
Kim J, Benton JF, Wisniewski D (2002) Pool boiling heat transfer on small heaters: effect of gravity and subcooling. Int J Heat Mass Transf 45(19):3921–3934
Lee HS, Merte H (1998) Pool boiling phenomena in microgravity. In: Proceedings of the 11th international heat transfer conference, Kyongju, Korea 2:395–400
Lienhard JH, Dhir VK (1973) Extended hydrodynamic theory of the peak and minimum pool boiling heat fluxes, NASA CR-2270
Marek H, Straub J (2001) The origin of thermocapillary convection in subcooled nucleate pool boiling. Int J Heat Mass Transf 44:629–632
McAdams WH (1954) Heat Transmission, 3rd edn., McGraw-Hill, New York, Chapter 7
McGrew JL, Bamford TR (1966) Marangoni flow: an additional mechanism in boiling heat transfer. Science 153(3740):1106–1107
Merte H, Lee, HS, Keller RB (1998) Dryout and rewetting in the pool boiling experiment flown on STS-72 (PBE-IIB) and STS-77 (PBE-IIA). NASA/CR–1998–207410
Merte H Jr, Lee HS, Keller RB (1996) Report on pool boiling experiment flown on STS-47 (PBE-IA), STS-57 (PBE-IB) and STS-60 (PBE-IC). NASA Contractor Report 198465
Ohta (2003) Review of reduced gravity boiling heat transfer: Japanese research. Jpn Soc Microgravity Appl J 20(4):272–285
Ostrach S (1982) Natural convection heat transfer in cavities and cells. In: Proceedings 7th international heat transfer conference, Vol. 1, pp 365–379
Raake D, Siekmann J (1989) Temperature and velocity fields due to surface tension driven flow. Exp Fluid 7:164–172
Rainey KN, You SM, Lee S (2003) Effect of pressure, subcooling, and dissolved gas on pool boiling heat transfer from microporous surfaces in FC-72. ASME J Heat Transf 125(1):75–83
Rosenhow WM (1962) A method of correlating heat transfer data for surface boiling of liquids. Trans ASME 84:969
Steinbichler M, Micko S, Straub J (1998) Nucleate boiling heat transfer on a small hemispherical heater and a wire under microgravity. In: Proceedings of the 11th international heat transfer conference, Kyongju, Korea, Vol. 2, pp 539–544
Straub J (2002) Origin and effect of thermocapillary convection in subcooled boiling: observations and conclusions from experiments performed at microgravity. Ann N Y Acad Sci 974:348–363
Straub J, Picker G, Winter J, Zell M (1997) Effective cooling of electronic components by boiling phase transition in microgravity. Acta Astronaut 40(2–8):119–127
Sun CI, Carey VP (2003) Effects of gap geometry and gravity on boiling around a constrained bubble in 2-propanol/water mixtures. In: Proceedings of the 2003 ASME summer heat transfer conference, Las Vegas
Trefethen L (1961) On the jet propulsion of bubbles in a heater liquid. Tufts University Mechanical Engineering Report, 61-8-1
Viskanta R et al (2000) Microgravity research in support of technologies for the human exploration and development of space and planetary bodies. Topical Report of the National Research Council–Space Studies Board
You SM, Simon TW, Bar-Cohen A, Hong YS (1995) Effects of dissolved gas content on pool boiling of a highly-wetting fluid. J Heat Transf 117(3):687–692
Wang H, Peng XF, Christopher DM, Lin WK, Pan C (2005) Investigation of bubble-top jet flow during subcooled boiling on wires. Int J Heat Fluid Flow 26:485–494
Zuber N (1959) Hydrodynamic aspects of boiling heat transfer. AEC report AECU-4439, Physics and Mathematics
Acknowledgments
This work was supported by the Human Support Research and Technology Division at NASA Headquarters, Grant No. NNC04AA26A.
Author information
Authors and Affiliations
Corresponding author
Rights and permissions
About this article
Cite this article
Henry, C.D., Kim, J. & McQuillen, J. Dissolved gas effects on thermocapillary convection during boiling in reduced gravity environments. Heat Mass Transfer 42, 919–928 (2006). https://doi.org/10.1007/s00231-006-0140-8
Received:
Accepted:
Published:
Issue Date:
DOI: https://doi.org/10.1007/s00231-006-0140-8